SAMPLING STRATEGIES FOR AQUATIC ENVIRONMENTS
Advice Note
Ideal sampling strategies will vary among habitats and monitoring contexts. We recommend that you speak to a member of our team to plan your survey. However, below is some guidance synthesized from eDNA research to date.
Note that if you are using bacterial kits, much less water is required to capture the bacterial community. You may choose to collect less water, and take fewer samples, than for other tests and kits.
Kit contents
Vampire Pump

1 x pump head (A)
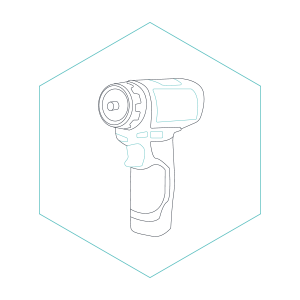
1 x drive unit (B)
PONDS
In a pond, eDNA does not mix well due to absence of flow or wave action (1-3), so multiple water samples are key to capture the eDNA present (4-6). A pond can be sampled for eDNA in two ways: 1) independent samples, each comprised of subsamples (e.g. 3 L comprised of 12 x 250 mL subsamples), can be taken at multiple locations around the pond perimeter (6-8), or 2) subsamples can be taken at multiple locations and pooled into a single composite sample (e.g. 3 L comprised of 30 x 100 mL subsamples) representing the entire pond (1,9).
Independent samples each passed through a separate filter will likely have higher detection rates as eDNA is more likely to be captured in at least one of the samples (6,10). Composite samples will reduce cost and allow more ponds to be sampled (1,10), but DNA from rare or low-density species may not be detected (7,8). Several composite samples may be needed for adequate eDNA representation from larger ponds (1).
When sampling ponds:
- Surface water (sub)samples should be collected from the shoreline at roughly equidistant intervals or targeting preferred habitat (if detection of a particular species is a priority) around the pond perimeter. (4,11)
- Use the provided sampling bag or ladle, or a clean bottle (we recommend a small mineral water bottle with the water discarded), to collect water. Deposit the collected (sub)sample in the provided sampling bag. Repeat for each subsample if applicable. Seal the bag and make sure the water is well mixed by shaking for 20-30 seconds. The bag is not self-standing, but can be supported by the surveyor or propped against a log, tree stump or rock to stabilize it for filtration.
- You should use a new bag/ladle/bottle for independent samples and for each pond to avoid cross-contamination (4,11).
- Depending on water clarity, it may be possible to pass up to 3 L of pond water through each filter used, but smaller volumes (e.g. 150-250 mL) are more typical for turbid ponds or ponds with dense vegetation (4,11).
LAKES
In a lake, eDNA can still be localized, so multiple water samples remain key to capture the eDNA present (4, 12-18). We recommend at least 10 independent samples, each comprised of subsamples (e.g. 3 L comprised of 12 x 250 mL subsamples), are taken from multiple locations around the lake perimeter (19,20). You should consider collecting one independent sample (i.e. using 1 kit) along 120 m of shoreline (maximum of shoreline), but contact NatureMetrics to discuss alternative sampling strategies to suit all budgets.
When sampling lakes:
- Surface water samples should be collected from the shoreline at roughly equidistant intervals around the lake perimeter (4).
- Use the provided sampling bag or ladle, or a clean bottle (we recommend a small mineral water bottle with the water discarded), to scoop up water every 10 m along a 120 m stretch of shoreline. Deposit the collected subsample in the provided sampling bag. Repeat for each subsample, seal the bag, and make sure the water is well mixed by shaking the bag for 20-30 seconds. The bag is not self-standing, but can be supported by the surveyor or propped against a log, tree stump or rock to stabilize it for filtration.
- Depending on water clarity, it may be possible to pass up to 3 L of lake water through each filter used, but smaller volumes (e.g. 2 L) are more typical (4).
- Sampling when the lake is not thermally stratified is ideal as more mixing of the water will occur. This means there is a higher chance of detecting eDNA from shallow and deep-water aquatic species (4,15,17,21). However, detection of invertebrates and some other taxonomic groups is generally lower in colder temperatures (22-25). In some cases, it is advisable to sample by boat and at varying depths to maximize detection rates (4,15,17,18,21). Please contact NatureMetrics to discuss your sampling plans.
RIVERS OR STREAMS
In a river or stream, eDNA can be well-mixed depending on local environmental conditions (26-30), but flow means that eDNA can be transported hundreds to thousands of meters from its source(29,31,32), and be diluted (27,33). As such, multiple sampling locations along the length of the stream/river are recommended (4). In small streams or rivers, five sampling locations should be identified, unless they are being considered as part of a broader catchment. In larger rivers, 20-60 sampling locations should be identified for a comprehensive survey (30,31,34-36).
At each sampling location, collect 3 L of water and filter through a single kit. To maximize and assess the likelihood of species detection, sampling can be split into three independent samples (kits) spanning the width of the stream/river section (e.g. separate kits for the left bank, center, and right bank) (26,34,37,38). If your budget does not allow for independent samples, subsamples at each sampling location are an alternative method to increase species detection (e.g. 1 L from the left bank, center, and right bank, pooled to give 3 L) (38-40). If sub-sampling is not feasible, alternate sampling locations between both sides of the stream/river as much as possible to account for variable eDNA distribution (27). For wide rivers, fast flowing rivers, and rivers with a large catchment, more water samples may be needed at each sampling location (26,34,37,38). We recommend speaking to our survey design team to help plan your survey in these instances or when a specific project question is being considered.
When sampling rivers or streams:
- Start at the most downstream sampling location and work your way upstream (4).
- Use of a sampling vessel/device and sampling from the shoreline is recommended. If shoreline sampling is not possible, surveyors should enter the water downstream of where they will collect the sample and be careful not to disturb sediment as they move to the collection point (4). If the water is too deep to enter, a boat or similar should be used for sampling.
- Use the provided sampling bag or ladle, or a clean bottle (we recommend a mineral water bottle with the water discarded), to collect water by holding it with the opening pointing upstream at the water surface. Stand downstream of the sampling bag/ladle/bottle to avoid collecting your own DNA. Deposit the collected sample in the provided sampling bag. Repeat for each sample, seal the bag, and make sure the water is well mixed by shaking for 20-30 seconds. The bag is not self-standing, but can be supported by the surveyor or propped against a log, tree stump or rock to stabilize it for filtration.
- Depending on water clarity, it may be possible to pass up to 3 L of stream/river water through each filter used, but smaller volumes (e.g. 2 L) are more typical.
ESTUARIES, SEAS OR OCEANS
In marine waterbodies, less is currently known about how hydrological systems affect eDNA transportation and distribution. However, it has been shown that communities obtained from marine eDNA metabarcoding are highly representative of the immediate local habitat where the sample was collected, both on horizontal and vertical planes (41-50) and across short timescales (47,21,52). This means that when samples are collected in a transect going from shore to offshore, different communities will be detected, sometimes even within the range of tens of metres (41,44,48,53). As in lakes, vertical stratification of the water (as a result of thermoclines) restricts mixing of eDNA, meaning that water samples should be collected from each depth zone of interest to fully characterize the marine communities at the sampling location (4,44,48,53,54).
eDNA in marine systems is generally much more dilute compared to that in freshwater systems (4,55). This is in part dependent on the target group or species but is particularly pertinent for larger vertebrates (extra-organismal vertebrate eDNA) (56,57). Planktonic or microbial taxa usually require smaller volumes (4). Therefore, sample volume should be maximized to be representative of the environment and the taxa that are targeted (58). Each sample should be at least 2 L volume (59), and the volume of water filtered should be in the range of 2-5 L (more is always better) (4,60). Turbidity is usually less of a problem in marine water, although inshore areas (e.g. mangrove forests, marinas, areas with high population density) can become turbid due to coastal run-off and wave action disturbing the sea floor (4,35). In this case, filter as much water as possible from each sample until the filter completely clogs.
Sample number will depend on the spatial scale of the study or monitoring project (4). In order to characterize a community or compare sites, a minimum of 20 samples, even for relatively small areas, is strongly advised (58). This usually involves collecting independent samples (rather than subsamples) spread out across the sampling area (4).
Sampling design will equally be dependent on the size of the sampling area, and also on the type of ecosystem (coral reef, mangrove forest, pelagic, etc.) that is targeted. Season (and even time of day) may need to be taken into consideration, as many fish species move to (in)shore areas for mating and spawning and move to deeper or warmer waters in winter, while other species may prefer cold, deep water during summer (15,61). Furthermore, some fish species exhibit diel migration where they move to a different position in the water column during the day or night which is reflected by eDNA analysis (52,54,62). Thus, it is important to consider migration patterns as well as mating and spawning sites.
When sampling estuaries, seas or oceans:
- Sampling is most often done from a boat, but samples may also be collected from the shoreline (4).
- Use the provided sampling bag or a Kemmerer/Niskin sampler to collect a minimum of 2 L of sea water per sample (59). Contact NatureMetrics if you do not have access to a Kemmerer/Niskin sampler.
- When using the Kemmerer/Niskin sampler to collect water near the bottom, be careful not to disturb the sediment as sediment present in water samples can clog filters and may contain ‘old’ eDNA that can skew inferences of species’ presence (63).
- You can use a manual syringe to filter the water, but peristaltic or vacuum pumps are more commonly used for larger volume samples (64).
- We recommend filtering 5 L (60), but the actual volume filtered will depend on the clarity and turbidity of the water (35). We suggest filtering no less than 2 L.
TO REPLICATE OR NOT TO REPLICATE?
Independent versus composite samples have been discussed as sampling strategies for several aquatic environments. Independent samples are field replicates, whether of an entire waterbody (e.g. pond, lake) or of a particular location in a waterbody (e.g. streams, rivers, seas, oceans). They add spatial context to the biological data obtained using eDNA analysis as each sample has its own coordinates (4). They also increase the probability of detecting species, especially those that are rare and/or present at low density, as eDNA is more likely to be captured (4,60,65–67). As composite samples are comprised of subsamples taken from multiple, distinct points around or within a waterbody that are pooled together, spatial resolution is lost (4) and eDNA of some species may be diluted by additional water (1,14,18), but there is more budget available to sample more locations and/or waterbodies (4,50). Which strategy to use ultimately depends on project aims, questions and budget (4,11).
If the question is simply ‘what species might be present in my study area?’, rare and/or low density species are of less concern, and statistical modelling is not required, then a composite sampling strategy will suffice. For example, one composite sample could be taken at each of 20 sampling locations along a river or multiple rivers as opposed to three independent samples at each sampling location, meaning 60 sampling locations could be surveyed instead of 20 sampling locations with the same budget.
If the question is more complex, such as ‘where do detected species occur?’, ‘what is the total number of species present in my study area?’ or ‘what is the error margin around species detection?’, then replicates are required to provide statistical power for models that perform predictive mapping, interpolate and extrapolate species richness, and estimate species detection probability or likelihood of false positives (i.e. species detected that is not actually present) and false negatives (i.e. species not detected that is actually present) (4,50,60,65–69). Please contact NatureMetrics to discuss your sampling plans if you have a particular question in mind.
CONTROLS
NatureMetrics recommend the inclusion of field negative controls (blanks) to detect possible contamination introduced during sampling. These are purified water (i.e. mineral, deionized or MilliQ water) processed in the same way as water samples (4,63,64,70).
The purified water should be poured into the sampling bag provided in the kit being used as a field blank. The bag should be sealed and shaken, then the purified water filtered as you would filter a natural water sample. Generally, filtering 500 mL of purified water is sufficient to serve as a field blank.
Once at the NatureMetrics laboratories, the field blank will be processed alongside your samples and screened with the analyses you selected to identify whether any contamination has occurred in the field. NatureMetrics Aquatic eDNA Kits contain only sterile, single-use components so no contamination is present before the kit is opened. However, contamination can be introduced by the surveyor as water samples are collected and filtered.
We recommend including at least one field blank at the end of each day that water sampling occurs, but ideally a field blank should be included for each waterbody sampled (70). NatureMetrics provide a free kit to be used as a field blank if 20 or more Aquatic eDNA Kits are purchased.
DECONTAMINATION
If you will be entering the water to collect samples, boots or waders should be decontaminated with bleach (containing sodium hypochlorite) in between waterbodies (4,11,63,64). Bleach should be disposed of responisibly. Please contact NatureMetrics for advice on appropriate decontamination procedures.
REFERENCES
1. Brys R, Haegeman A, Halfmaerten D, Neyrinck S, Staelens A, Auwerx J, et al. Monitoring of spatiotemporal occupancy patterns of fish and amphibian species in a lentic aquatic system using environmental DNA. Mol Ecol [Internet]. 2021 Jul;30(13):3097–110. Available from: http://dx.doi.org/10.1111/mec.15742
2. Li J, Lawson Handley LJ, Harper LR, Brys R, Watson HV, Di Muri C, et al. Limited dispersion and quick degradation of environmental DNA in fish ponds inferred by metabarcoding. Environmental DNA [Internet]. 2019 Jun 25;5:133. Available from: http://doi.wiley.com/10.1002/edn3.24
3. Shu L, Chen S, Li P, Peng Z. Environmental DNA Metabarcoding Reflects Fish DNA Dynamics in Lentic Ecosystems: A Case Study of Freshwater Ponds. Fish Sahul [Internet]. 2022 Sep 26 [cited 2022 Oct 2];7(5):257. Available from: https://www.mdpi.com/2410-3888/7/5/257
4. Bruce K, Blackman R, Bourlat SJ, Hellström AM, Bakker J, Bista I, et al. A practical guide to DNA-based methods for biodiversity assessment. AB [Internet]. 2021 Feb 12;1:Advanced Books. Available from: https://doi.org/10.3897/ab.e68634
5. Goldberg CS, Strickler KM, Fremier AK. Degradation and dispersion limit environmental DNA detection of rare amphibians in wetlands: Increasing efficacy of sampling designs. Sci Total Environ [Internet]. 2018 Mar 27;633:695–703. Available from: http://dx.doi.org/10.1016/j.scitotenv.2018.02.295
6. Harper LR, Handley LL, Carpenter AI, Ghazali M, Di Muri C, Macgregor CJ, et al. Environmental DNA (eDNA) metabarcoding of pond water as a tool to survey conservation and management priority mammals. Biol Conserv [Internet]. 2019 [cited 2019 Sep 3];238:108225. Available from: http://dx.doi.org/10.1016/j.biocon.2019.108225
7. Blabolil P, Griffiths NP, Hänfling B, Jůza T, Draštík V, Knežević-Jarić J, et al. The true picture of environmental DNA, a case study in harvested fishponds. Ecol Indic [Internet]. 2022 Sep 1;142:109241. Available from: https://www.sciencedirect.com/science/article/pii/S1470160X22007130
8. Di Muri C, Lawson Handley L, Bean CW, Li J, Peirson G, Sellers GS, et al. Read counts from environmental DNA (eDNA) metabarcoding reflect fish abundance and biomass in drained ponds. MBMG [Internet]. 2020 Oct 22;4:e56959. Available from: https://doi.org/10.3897/mbmg.4.56959
9. Biggs J, Ewald N, Valentini A, Gaboriaud C, Dejean T, Griffiths RA, et al. Using eDNA to develop a national citizen sciencebased monitoring programme for the great crested newt (Triturus cristatus). Biol Conserv [Internet]. 2015;183:19–28. Available from: http://www.sciencedirect.com/science/article/pii/S0006320714004546
10. Davis AJ, Williams KE, Snow NP, Pepin KM, Piaggio AJ. Accounting for observation processes across multiple levels of uncertainty improves inference of species distributions and guides adaptive sampling of environmental DNA. Ecol Evol [Internet]. 2018 Nov;8(22):10879–92. Available from: http://dx.doi.org/10.1002/ece3.4552
11. Harper LR, Buxton AS, Rees HC, Bruce K, Brys R, Halfmaerten D, et al. Prospects and challenges of environmental DNA (eDNA) monitoring in freshwater ponds. Hydrobiologia [Internet]. 2019;826(1):25–41. Available from: https://doi.org/10.1007/s10750-018-3750-5
12. Eichmiller JJ, Bajer PG, Sorensen PW. The relationship between the distribution of common carp and their environmental DNA in a small lake. PLoS One [Internet]. 2014 Nov 10;9(11):e112611. Available from:
http://dx.doi.org/10.1371/journal.pone.0112611
13. Hänfling B, Lawson Handley L, Read DS, Hahn C, Li J, Nichols P, et al. Environmental DNA metabarcoding of lake fish communities reflects long-term data from established survey methods. Mol Ecol [Internet]. 2016 Apr 20;25:3101–19. Available from: http://dx.doi.org/10.1111/mec.13660
14. Sato H, Sogo Y, Doi H, Yamanaka H. Usefulness and limitations of sample pooling for environmental DNA metabarcoding of freshwater fish communities. Sci Rep [Internet]. 2017 Nov 1;7(1):14860. Available from: http://dx.doi.org/10.1038/s41598-017-14978-6
15. Lawson Handley L, Read DS, Winfield IJ, Kimbell H, Johnson H, Li J, et al. Temporal and spatial variation in distribution of fish environmental DNA in England’s largest lake. Environmental DNA [Internet]. 2019 Apr 15;1(1):26–39. Available from: https://onlinelibrary.wiley.com/doi/abs/10.1002/edn3.5
16. Di Muri C, Lawson Handley L, Bean CW, Benucci M, Harper LR, James B, et al. Spatio‐temporal monitoring of lake fish spawning activity using environmental DNA metabarcoding. Environmental DNA [Internet]. 2022 Jul 26; Available from: https://onlinelibrary.wiley.com/doi/10.1002/edn3.343
17. Hervé A, Domaizon I, Baudoin J-M, Dejean T, Gibert P, Jean P, et al. Spatio-temporal variability of eDNA signal and its implication for fish monitoring in lakes. PLoS One [Internet]. 2022 Aug 12;17(8):e0272660. Available from:
http://dx.doi.org/10.1371/journal.pone.0272660
18. Zhang S, Lu Q, Wang Y, Wang X, Zhao J, Yao M. Assessment of fish communities using environmental DNA: Effect of spatial sampling design in lentic systems of different sizes. Mol Ecol Resour [Internet]. 2020 Jan;20(1):242–55. Available from: http://dx.doi.org/10.1111/1755-0998.13105
19. Li J, Hatton-Ellis TW, Lawson Handley L-J, Kimbell HS, Benucci M, Peirson G, et al. Ground-truthing of a fish-based environmental DNA metabarcoding method for assessing the quality of lakes. Journal of Applied Ecology [Internet]. 2019 Feb 3;56(5):1232–44. Available from: http://doi.wiley.com/10.1111/1365-2664.13352
20. Bedwell ME, Goldberg CS. Spatial and temporal patterns of environmental DNA detection to inform sampling protocols in lentic and lotic systems. Ecol Evol [Internet]. 2020 Feb;10(3):1602–12. Available from: http://dx.doi.org/10.1002/ece3.6014
21. Littlefair JE, Hrenchuk LE, Blanchfield PJ, Rennie MD, Cristescu ME. Thermal stratification and fish thermal preference explain vertical eDNA distributions in lakes. Mol Ecol [Internet]. 2020 Sep 5; Available from:
http://dx.doi.org/10.1111/mec.15623
22. Schmidt KJ, Soluk DA, Maestas SEM, Britten HB. Persistence and accumulation of environmental DNA from an endangered dragonfly. Sci Rep [Internet]. 2021 Sep 23 [cited 2021 Sep 28];11(1):18987. Available from:
https://www.nature.com/articles/s41598-021-98099-1
23. Reinholdt Jensen M, Egelyng Sigsgaard E, Agersnap S, Jessen Rasmussen J, Baattrup‐Pedersen A, Wiberg‐Larsen P, et al. Seasonal turnover in community composition of stream‐associated macroinvertebrates inferred from freshwater
environmental DNA metabarcoding. Environmental DNA [Internet]. 2021 Mar 26;(edn3.193). Available from: https://onlinelibrary.wiley.com/doi/10.1002/edn3.193
24. Harper K, Anucha P, Turnbull J, Bean C, Leaver M. Searching for a signal: Environmental DNA (eDNA) for the detection of invasive signal crayfish, Pacifastacus leniusculus (Dana, 1852). Management of Biological Invasions [Internet]. 2018;9(2):137–48. Available from: http://www.reabic.net/journals/mbi/2018/Issue2.aspx
25. Troth CR, Sweet MJ, Nightingale J, Burian A. Seasonality, DNA degradation and spatial heterogeneity as drivers of eDNA detection dynamics. Sci Total Environ [Internet]. 2021 May 10;768:144466. Available from: http://dx.doi.org/10.1016/j.scitotenv.2020.144466
26. Laporte M, Berger CS, García-Machado E, Côté G, Morissette O, Bernatchez L. Cage transplant experiment shows weak transport effect on relative abundance of fish community composition as revealed by eDNA metabarcoding. Ecol Indic [Internet]. 2022 Apr 1;137:108785. Available from: https://www.sciencedirect.com/science/article/pii/S1470160X22002564
27. Thalinger B, Kirschner D, Pütz Y, Moritz C, Schwarzenberger R, Wanzenböck J, et al. Lateral and longitudinal fish environmental DNA distribution in dynamic riverine habitats. Environmental DNA [Internet]. 2021 Jan;3(1):305–18. Available from: https://onlinelibrary.wiley.com/doi/10.1002/edn3.171
28. Berger CS, Hernandez C, Laporte M, Côté G, Paradis Y, Kameni T. DW, et al. Fine‐scale environmental heterogeneity shapes fluvial fish communities as revealed by eDNA metabarcoding. Environmental DNA [Internet]. 2020 Aug 20;(edn3.129). Available from: https://onlinelibrary.wiley.com/doi/10.1002/edn3.129
29. Spence BC, Rundio DE, Demetras NJ, Sedoryk M. Efficacy of environmental DNA sampling to detect the occurrence of endangered coho salmon (Oncorhynchus kisutch ) in Mediterranean‐climate streams of California’s central coast. Environmental DNA [Internet]. 2021 Jul;3(4):727–44. Available from: https://onlinelibrary.wiley.com/doi/10.1002/edn3.175
30. Cantera I, Decotte J-B, Dejean T, Murienne J, Vigouroux R, Valentini A, et al. Characterizing the spatial signal of environmental DNA in river systems using a community ecology approach. Mol Ecol Resour [Internet]. 2021 Nov 1; Available from: http://dx.doi.org/10.1111/1755-0998.13544
31. Pont D, Rocle M, Valentini A, Civade R, Jean P, Maire A, et al. Environmental DNA reveals quantitative patterns of fish biodiversity in large rivers despite its downstream transportation. Sci Rep [Internet]. 2018 Jul 10;8(1):10361. Available from: http://dx.doi.org/10.1038/s41598-018-28424-8
32. Wacker S, Fossøy F, Larsen BM, Brandsegg H, Sivertsgård R, Karlsson S. Downstream transport and seasonal variation in freshwater pearl mussel (Margaritifera margaritifera ) eDNA concentration. Environmental DNA [Internet]. 2019 May 16;1(1):64–73. Available from: https://onlinelibrary.wiley.com/doi/abs/10.1002/edn3.10
33. Curtis AN, Tiemann JS, Douglass SA, Davis MA, Larson ER. High stream flows dilute environmental DNA (eDNA) concentrations and reduce detectability. Divers Distrib [Internet]. 2020 Nov 14;(ddi.13196). Available from: http://dx.doi.org/10.1111/ddi.13196
34. Broadhurst HA, Gregory LM, Bleakley EK, Perkins JC, Lavin JV, Bolton P, et al. Mapping differences in mammalian distributions and diversity using environmental DNA from rivers. Sci Total Environ [Internet]. 2021 Dec 20;801:149724. Available from: https://www.sciencedirect.com/science/article/pii/S0048969721047999
35. Hallam J, Clare EL, Jones JI, Day JJ. Biodiversity assessment across a dynamic riverine system: A comparison of eDNA metabarcoding versus traditional fish surveying methods. Environmental DNA [Internet]. 2021 Jul 21;(edn3.241). Available from: https://onlinelibrary.wiley.com/doi/10.1002/edn3.241
36. Carraro L, Mächler E, Wüthrich R, Altermatt F. Environmental DNA allows upscaling spatial patterns of biodiversity in freshwater ecosystems. Nat Commun [Internet]. 2020 Jul 17;11(1):3585. Available from: http://dx.doi.org/10.1038/s41467-020-17337-8
37. Bylemans J, Gleeson DM, Lintermans M, Hardy CM, Beitzel M, Gilligan DM, et al. Monitoring riverine fish communities through eDNA metabarcoding: determining optimal sampling strategies along an altitudinal and biodiversity gradient. Metabarcoding and Metagenomics [Internet]. 2018;2:e30457. Available from: https://mbmg.pensoft.net/article/30457/download/pdf/
38. Cantera I, Cilleros K, Valentini A, Cerdan A, Dejean T, Iribar A, et al. Optimizing environmental DNA sampling effort for fish inventories in tropical streams and rivers. Sci Rep [Internet]. 2019 Feb 28;9(1):3085. Available from:
http://dx.doi.org/10.1038/s41598-019-39399-5
39. Mächler E, Deiner K, Spahn F, Altermatt F. Fishing in the water: effect of sampled water volume on environmental DNA-based detection of macroinvertebrates. Environ Sci Technol [Internet]. 2015 Nov 11; Available from: http://dx.doi.org/10.1021/acs.est.5b04188
40. Lopes CM, Sasso T, Valentini A, Dejean T, Martins M, Zamudio KR, et al. eDNA metabarcoding: a promising method for anuran surveys in highly diverse tropical forests. Mol Ecol Resour [Internet]. 2016 Dec 16;17:904–14. Available from: http://dx.doi.org/10.1111/1755-0998.12643
41. Port JA, O’Donnell JL, Romero-Maraccini OC, Leary PR, Litvin SY, Nickols KJ, et al. Assessing vertebrate biodiversity in a kelp forest ecosystem using environmental DNA. Mol Ecol [Internet]. 2016 Jan;25(2):527–41. Available from: http://dx.doi.org/10.1111/mec.13481
42. Yamamoto S, Masuda R, Sato Y, Sado T, Araki H, Kondoh M, et al. Environmental DNA metabarcoding reveals local fish communities in a species-rich coastal sea. Sci Rep [Internet]. 2017 Jan 12;7:40368. Available from:http://dx.doi.org/10.1038/srep40368
43. Jeunen G-J, Knapp M, Spencer HG, Lamare MD, Taylor HR, Stat M, et al. Environmental DNA (eDNA) metabarcoding reveals strong discrimination among diverse marine habitats connected by water movement. Mol Ecol Resour [Internet]. 2019 Mar;19(2):426–38. Available from: http://dx.doi.org/10.1111/1755-0998.12982
44. Jeunen G, Lamare MD, Knapp M, Spencer HG, Taylor HR, Stat M, et al. Water stratification in the marine biome restricts vertical environmental DNA (eDNA) signal dispersal. Environmental DNA [Internet]. 2020 Jan 13;2(1):99–111. Available from: https://onlinelibrary.wiley.com/doi/abs/10.1002/edn3.49
45. Djurhuus A, Closek CJ, Kelly RP, Pitz KJ, Michisaki RP, Starks HA, et al. Environmental DNA reveals seasonal shifts and potential interactions in a marine community. Nat Commun [Internet]. 2020 Jan 14;11(1):254. Available from: http://dx.doi.org/10.1038/s41467-019-14105-1
46. West KM, Stat M, Harvey ES, Skepper CL, DiBattista JD, Richards ZT, et al. eDNA metabarcoding survey reveals fine-scale coral reef community variation across a remote, tropical island ecosystem. Mol Ecol [Internet]. 2020 Mar;29(6):1069–86. Available from: http://dx.doi.org/10.1111/mec.15382
47. Ely T, Barber PH, Man L, Gold Z. Short-lived detection of an introduced vertebrate eDNA signal in a nearshore rocky reef environment. PLoS One [Internet]. 2021 Jun 4;16(6):e0245314. Available from: http://dx.doi.org/10.1371/journal.pone.0245314
48. Monuki K, Barber PH, Gold Z. eDNA captures depth partitioning in a kelp forest ecosystem. PLoS One [Internet]. 2021 Nov 4;16(11):e0253104. Available from: http://dx.doi.org/10.1371/journal.pone.0253104
49. Lafferty KD, Garcia‐Vedrenne AE, McLaughlin JP, Childress JN, Morse MF, Jerde CL. At Palmyra Atoll , the fish‐community environmental DNA signal changes across habitats but not with tides. J Fish Biol [Internet]. 2020 Jun 29;1. Available from:
https://onlinelibrary.wiley.com/doi/abs/10.1111/jfb.14403
50. Larson WA, Barry P, Dokai W, Maselko J, Olson J, Baetscher D. Leveraging eDNA metabarcoding to characterize nearshore fish communities in Southeast Alaska: Do habitat and tide matter? Environmental DNA [Internet]. 2022 Mar 29; Available from: https://onlinelibrary.wiley.com/doi/10.1002/edn3.297
51. Murakami H, Yoon S, Kasai A, Minamoto T, Yamamoto S, Sakata MK, et al. Dispersion and degradation of environmental DNA from caged fish in a marine environment. Fish Sci [Internet]. 2019 Jan 3; Available from: https://doi.org/10.1007/s12562-018-1282-6
52. Jensen MR, Sigsgaard EE, Ávila M de P, Agersnap S, Brenner-Larsen W, Sengupta ME, et al. Short‐term temporal variation of coastal marine eDNA. Environmental DNA [Internet]. 2022 Feb 23; Available from: https://onlinelibrary.wiley.com/doi/10.1002/edn3.285
53. Allan EA, DiBenedetto MH, Lavery AC, Govindarajan AF, Zhang WG. Modeling characterization of the vertical and temporal variability of environmental DNA in the mesopelagic ocean. Sci Rep [Internet]. 2021 Oct 28;11(1):21273. Available from: http://dx.doi.org/10.1038/s41598-021-00288-5
54. Canals O, Mendibil I, Santos M, Irigoien X, Rodríguez‐Ezpeleta N. Vertical stratification of environmental DNA in the open ocean captures ecological patterns and behavior of deep‐sea fishes. Limnol Oceanogr Lett [Internet]. 2021 Sep 17; Available from: http://dx.doi.org/10.1002/lol2.10213
55. Harrison JB, Sunday JM, Rogers SM. Predicting the fate of eDNA in the environment and implications for studying biodiversity. Proc Biol Sci [Internet]. 2019 Nov 20;286(1915):20191409. Available from: http://dx.doi.org/10.1098/rspb.2019.1409
56. Suarez-Bregua P, Álvarez-González M, Parsons KM, Rotllant J, Pierce GJ, Saavedra C. Environmental DNA (eDNA) for monitoring marine mammals: Challenges and opportunities. Frontiers in Marine Science [Internet]. 2022;9. Available from: https://www.frontiersin.org/articles/10.3389/fmars.2022.987774
57. Harper KJ, Goodwin KD, Harper LR, LaCasella EL, Frey A, Dutton PH. Finding Crush: Environmental DNA Analysis as a Tool for Tracking the Green Sea Turtle Chelonia mydas in a Marine Estuary. Frontiers in Marine Science [Internet]. 2020;6:810. Available from: https://www.frontiersin.org/article/10.3389/fmars.2019.00810
58. Stauffer S, Jucker M, Keggin T, Marques V, Andrello M, Bessudo S, et al. How many replicates to accurately estimate fish biodiversity using environmental DNA on coral reefs? Ecol Evol [Internet]. 2021 Oct 6; Available from: https://onlinelibrary.wiley.com/doi/10.1002/ece3.8150
59. Bessey C, Jarman SN, Berry O, Olsen YS, Bunce M, Simpson T, et al. Maximizing fish detection with eDNA metabarcoding. Environmental DNA [Internet]. 2020 Feb 20;1:1. Available from: https://onlinelibrary.wiley.com/doi/abs/10.1002/edn3.74
60. Valsecchi E, Arcangeli A, Lombardi R, Boyse E, Carr IM, Galli P, et al. Ferries and Environmental DNA: Underway Sampling From Commercial Vessels Provides New Opportunities for Systematic Genetic Surveys of Marine Biodiversity. Frontiers in Marine Science [Internet]. 2021;8:1136. Available from: https://www.frontiersin.org/article/10.3389/fmars.2021.704786
61. Sigsgaard EE, Nielsen IB, Carl H, Krag MA, Knudsen SW, Xing Y, et al. Seawater environmental DNA reflects seasonality of a coastal fish community. Mar Biol [Internet]. 2017 Jun 1 [cited 2017 Nov 18];164(6):128. Available from:
https://link.springer.com/article/10.1007/s00227-017-3147-4
62. Easson CG, Boswell KM, Tucker N, Warren JD, Lopez JV. Combined eDNA and Acoustic Analysis Reflects Diel Vertical Migration of Mixed Consortia in the Gulf of Mexico. Frontiers in Marine Science [Internet]. 2020; Available from:
http://search.proquest.com/openview/4541335bdb380c55dce59e8589961be5/1?pq-origsite=gscholar&cbl=2049538
63. Goldberg CS, Turner CR, Deiner K, Klymus KE, Thomsen PF, Murphy MA, et al. Critical considerations for the application of environmental DNA methods to detect aquatic species. Methods Ecol Evol [Internet]. 2016 Nov 1;7(11):1299–307. Available from: http://dx.doi.org/10.1111/2041-210X.12595
64. Cowart DA, Murphy KR, Cheng C-HC. Environmental DNA from Marine Waters and Substrates: Protocols for Sampling and eDNA Extraction. In: Verde C, Giordano D, editors. Marine Genomics: Methods and Protocols [Internet]. New York, NY: Springer US; 2022. p. 225–51. Available from: https://doi.org/10.1007/978-1-0716-2313-8_11
65. Fukaya K, Kondo NI, Matsuzaki S-IS, Kadoya T. Multispecies site occupancy modeling and study design for spatially replicated environmental DNA metabarcoding. Methods Ecol Evol [Internet]. 2021 Oct 4;n/a(n/a). Available from: https://doi.org/10.1111/2041-210X.13732
66. Macher T-H, Schütz R, Arle J, Beermann AJ, Koschorreck J, Leese F. Beyond fish eDNA metabarcoding: Field replicates disproportionately improve the detection of stream associated vertebrate species. MBMG [Internet]. 2021 Jul 13;5:e66557. Available from: https://doi.org/10.3897/mbmg.5.66557
67. Ficetola GF, Pansu J, Bonin A, Coissac E, Giguet-Covex C, De Barba M, et al. Replication levels, false presences and the estimation of the presence/absence from eDNA metabarcoding data. Mol Ecol Resour [Internet]. 2015 May;15(3):543–56. Available from: http://dx.doi.org/10.1111/1755-0998.12338
68. Buxton A, Matechou E, Griffin J, Diana A, Griffiths RA. Optimising sampling and analysis protocols in environmental DNA studies. Sci Rep [Internet]. 2021 Jun 2;11(1):11637. Available from: http://dx.doi.org/10.1038/s41598-021-91166-7
69. Erickson RA, Merkes CM, Mize EL. Sampling Designs for Landscape‐level eDNA Monitoring Programs. Integr Environ Assess Manag [Internet]. 2019 Sep 7;15(5):760–71. Available from: https://onlinelibrary.wiley.com/doi/abs/10.1002/ieam.4155
70. Hutchins PR, Simantel LN, Sepulveda AJ. Time to get real with qPCR controls: The frequency of sample contamination and the informative power of negative controls in environmental (e)DNA studies. Mol Ecol Resour [Internet]. 2021 Nov 9; Available from: http://dx.doi.org/10.1111/1755-0998.1354